An artist’s rendering of a black hole
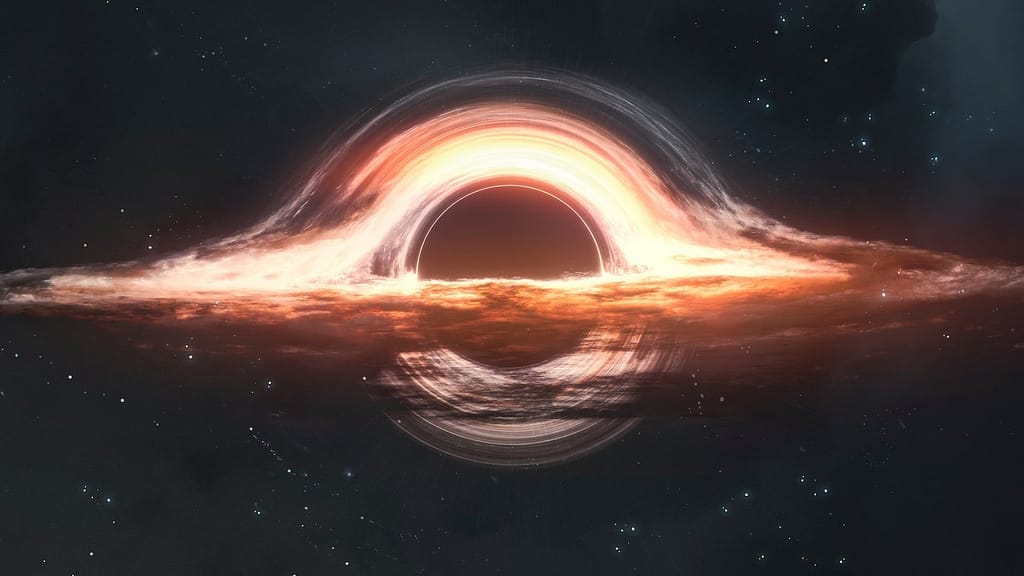
At the Milky Way’s core lies a gigantic enigma. Located in the center of many galaxies, supermassive black holes are enormous breaks in space-time that periodically absorb matter before spewing it out at speeds close to light, influencing the evolution of galaxies.
However, how they became so massive is still a question in astrophysics, and the James Webb Space Telescope (JWST) has added to the puzzle. The telescope, which has been in operation since 2022, has discovered that the cosmic monsters are remarkably enormous and numerous in the few million years following the Big Bang. This finding contradicts many of our best theories on the formation of black holes.
Astrophysicist Sophie Koudmani of the University of Cambridge is trying to find solutions to this issue. At London’s New Scientist Live event, Live Science spoke her about the cosmic monsters, their formation, and how her work simulating them with supercomputers might change the course of our universe’s history.
Ben Turner: What makes supermassive black holes so crucial to our knowledge of the cosmos?
Sophie Koudmani: Everything in the cosmos is interconnected, and supermassive black holes are crucial. The area surrounding the black holes provides them with a vast quantity of energy. The gravitational potential energy of the gas is transformed into radiation as it descends in. As a result, the gas becomes very hot and begins to shine.
The entire galaxy is impacted by the gas’s radiation once it has been heated to millions of degrees. In order to create realistic galaxies, it pauses star formation by preventing gas from clumping together to form stars. The large-scale structure of the universe can then be influenced by the energy [from supermassive black holes] traveling even farther out, which is crucial for cosmology and comprehending cosmic development.
BT: You mean relativistic jets, or outflows from some black holes that are close to the speed of light, when you talk about the energy going outwards, correct?
SK: In agreement. Black holes may “speak” to their host galaxies in three different ways. The accretion disk, which is the cloud-like structure of gas, dust, and plasma that circles black holes, emits winds that are not as thin as relativistic jets. Radiation is another method. Therefore, disks typically emit radiation from various electromagnetic spectrums as well as X-rays.
BT: I know you said this, but how would galaxies seem if there were no black holes?
SK: Accordingly, you can have what is commonly referred to as “runaway star formation.” You would receive balls of stars and the gas would be swiftly exhausted. Galaxies don’t look like this. It is crucial to have a black hole of some sort in order to obtain the disk galaxies that we observe in our universe. A realistic gas-to-star ratio must be achieved without the stars being immediately consumed.
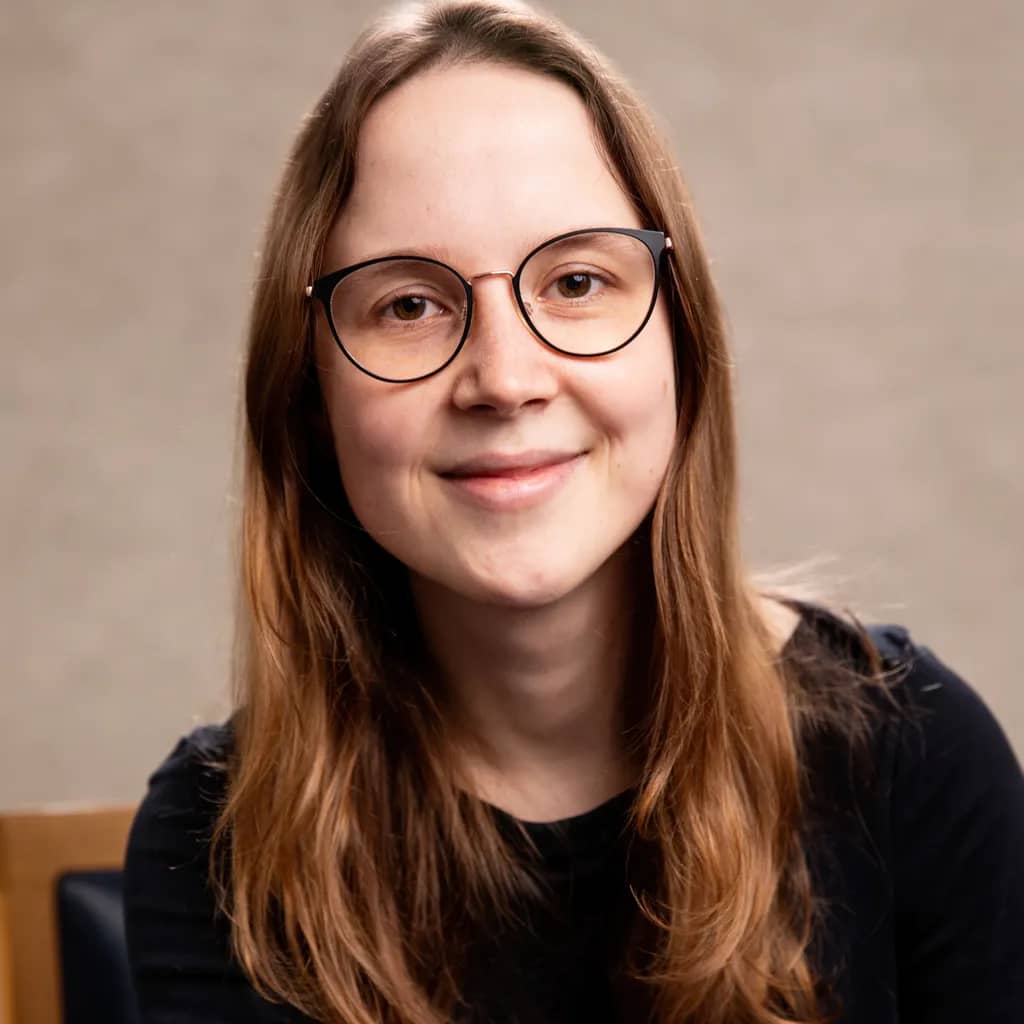
Why did you decide to research black holes, BT? What inquiries do you wish to address regarding them?
SK: One aspect of supermassive black holes that I find very appealing is their seeming simplicity, which is followed by their extraordinarily complicated physics. The “no hair theorem” states that you can describe black holes using just two numbers: their mass and spin. This tells you all you need to know about how they behave. All of these options may be obtained from these two integers. For instance, some black holes have jets, while others do not; some have accretion disks that shine brilliantly, while others are silent. This is brought forth by the contact with the galaxies.
Thus, it’s a basic item at its core that has the potential to be immensely potent. It interacts with a galaxy, which may be a chaotic and complex object. The gas, dust, and stars are all kept together by dark matter, which is a subject we don’t fully comprehend. Furthermore, it is quite difficult to comprehend how each of these elements interacts with the others.
BT: Relativistic physics is where all of our equations fall down, and it’s funny that you called them easy. This is where we might want to explore theories of quantum gravity. Do our theories about them make them appear simple?
SK: Depending on your interests, yes. Indeed, the singularity is where our ideas fail if you’re curious in what’s happening inside the event horizon. Other physical processes that could originate from inside the black hole, such as Hawking radiation, are unknown.
Yes, you have a really challenging job if you’re worried about all of this! However, the gas fluxes and radiation surrounding the black hole are of relevance if you’re considering astrophysical black holes. Locating the event horizon, seeing its effects on the surrounding area, and remaining mostly indifferent to its contents may be a source of great satisfaction for an astrophysicist. The mass and the spin influence the position of that horizon itself in a unique way.
BT: What previously unknown secrets concerning black holes have JWST uncovered?
SK: At such an early stage, we were unaware that there would be so many supermassive black holes. It was unexpected that they were found in such large quantities [in the early cosmos] and inside relatively tiny galaxies.
I was fortunate to be working on my PhD, which focused on simulating black holes in tiny galaxies, as this topic has become quite important for understanding the early universe. According to JWST, black hole activity occurred in more galaxies and at far earlier times than previously believed. The activity appears to be more efficient than in the modern cosmos, in fact.
BT: Why might that be?
SK: As everyone is aware, the universe expands after the Big Bang. This implies that in the early cosmos, everything was somewhat closer together, which led to larger gas inflows, which may have aided in the feeding of black holes.
One issue is that supernovae and black holes are somewhat in competition with one another. Black holes and star formation both use up gas. Both the black hole and the supernovae expel gas, and the supernovae also remove gas from the center area. As a result, black holes are unable to expand since the gas has been expelled. It’s possible that this doesn’t occur as frequently in the early cosmos for whatever reason, and the black hole just prevails in that process.
Indeed, there is a clear indication that [in the early cosmos], the black holes prevail. Given the size of these black holes, it virtually implies that they formed more quickly than their host galaxies.
BT: You brought up black hole efficiency as well. In other words, how can black holes be efficient?
SK: There are several methods. One method is to ask how strongly accreting [the rate at which the accretion disk increases] the gas is when they bring it in. A black hole speed limit known as the Eddington Limit exists. We frequently calculate the amount that the black hole is expanding by sucking in gas as a percentage of that theoretical upper limit. The efficiency of certain objects measured by the JWST is over 100%, indicating that they are incredibly efficient.
It also implies that it is not a hard limit because it is always based on theory and assumptions, some of which may be incorrect. Since they are able to surpass the limit and expand even more quickly, Webb has really demonstrated to us that they are obviously mistaken in those situations.
BT: So why does that efficiency decline as we get into the local universe, the latter phases of the universe?
SK: Therefore, there is only less gas there when there is greater star formation. As a result, galaxies may become increasingly gas impoverished, with some of that gas being ejected away, some becoming stars, and some being eaten up by black holes. Known as elliptical galaxies, very ancient galaxies are often dominated by their stars.
BT: First of all, how do black holes form? I take it that there are three main ways? Walk us through them.
SK: So, the first one is for the stars of the first generation. Therefore, they would have been almost 100 times as large as our sun. Black holes are created when they reach the end of their lives and collapse. Given that we’re starting at 100 [solar masses] and aim to reach 1 million, this might either be a favorable starting place [for supermassive black holes] or a difficult one.
Massive gas clouds would be a lot simpler place to start. It is considerably simpler to reach supermassive black holes [mass scales] since these collapse straight into black holes and begin at a mass of around 100,000 times that of the sun. An intermediate situation is nuclear star clusters, in which a large number of stars form in galaxies’ cores before collapsing into black holes.
BT: Another possibility is the existence of primordial black holes, which are thought to be remnants of a pre-Big Bang era. Is there much data to support this viewpoint, which is somewhat unconventional?
SK: That hypothesis is rather unconventional. More restrictions are being placed on it, but it isn’t completely ruled out. The intriguing thing about this topic at the moment, in my opinion, is that nothing is ruled out. As we approach the times at when these black holes created, the limitations become more stringent.
BT: How might we eliminate it at last? What limitations exist?
SK: Some claim that since huge black holes had been discovered so early in the cosmos, direct collapse must have produced them. According to a number of published articles, the observations support this.
In order to determine whether there are any additional possibilities for alternative theories, we are now updating our simulations of how black holes expanded in the early universe. Black holes have just enough time to form from a relatively light seed, especially if they expand effectively. The intriguing part, in my opinion, at the moment is that none of the models are ruled out.
BT: How are we searching for solutions, then? We’ve spoken about how the JWST discovered black holes earlier and earlier. Are there any additional avenues we’re looking at to learn more?
SK: Using gravitational waves is a pretty intriguing method. We’ll be able to map the population of supermassive black holes in a completely new way if we can find them. Because the only method to detect supermassive black holes at the moment is if they are in an active phase, unless a black hole is quite close to us and we are able to map out these star orbits.
However, we will have a second channel to assist us estimate their masses once we have gravitational wave equipment that can detect supermassive black hole mergers. And since these sensors would be extremely sensitive, it would go all the way back to the early cosmos. After that, we may identify merger indicators and identify workable growth strategies.
BT: Your task is to identify potential growth paths using simulations. How do they aid in our search for solutions?
SK: Simulation and observation are always interacting. Thus, an observation provides us with an explanation, such as the early supermassive black holes. Therefore, it may be necessary to modify models in order to accommodate such development at an early stage. We may then modify our models after the simulations have given us a better idea of what to search for.
In order to better comprehend these supermassive black holes, I work closely with observers and am a member of a big JWST program that will collect observations next year and do follow-ups of these young black holes.
BT: In conclusion, what fields of recent large black hole research are you most enthusiastic about?
SK: The gravitational wave detector LISA, which is scheduled to be online in the 2030s, excites me much since it will allow us to study gravity waves from supermassive black holes as well as tiny ones. To achieve it, you must be in space.
I’m also thrilled about technical development because I’m a bit of a nerd when it comes to coding and model construction. Naturally, artificial intelligence is a fascinating example that is frequently featured in the news.
AI is being used to speed up and improve the accuracy of our simulations while attempting to cross all scales, from event horizons to the vast expanse of the cosmic web. The processing resources of even the largest, most powerful supercomputers are too demanding to accomplish this directly at this time, but we can employ artificial intelligence (AI) to create solutions.